Astronomy Object of the Month: November 2024
< previous Archive next >
A New Effective Method for Analyzing Extremely Low-Frequency Electromagnetic Waves
Extremely low frequency electromagnetic waves (ELF; here from 0.03 to 1000 Hz) propagating in the spherical resonant cavity between the Earth's surface and the ionosphere provide an effective means of testing the ionosphere's state and its inhomogeneities. They allow for the analysis of solar activity impacts in the UV and X-ray range, solar flares, and space weather. Additionally, they enable studies of such terrestrial phenomena of importance as climate change. Analysis of ELF waves is also crucial for mitigating possible coherent electromagnetic interference in gravitational wave detector networks, like LIGO-VIRGO-KAGRA. Our new measurements from the ELA11 station in the Bieszczady Mountains offer promising potential for advancing such research. Here, we present the first in a series of studies conducted over the past year based on data from this station.
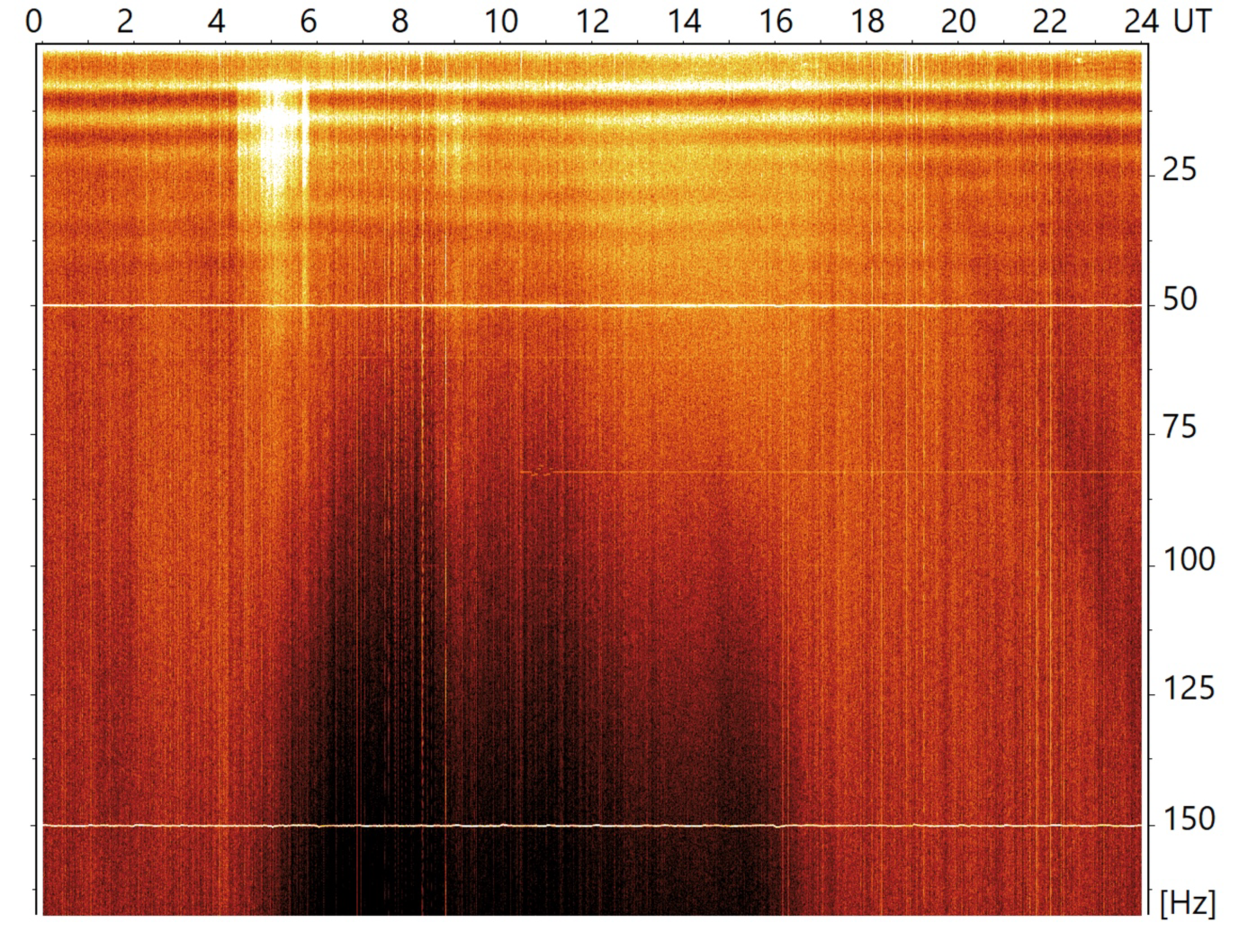
Electrical discharges accompanying thunderstorms generate strong ELF electromagnetic impulses, capable of circling the Earth multiple times within the spherical resonant cavity formed between the Earth's surface and the ionosphere. The interference of these waves creates maxima in the ELF spectrum, known as Schumann resonances, at frequencies of 8 Hz, 14 Hz, 20 Hz, and so on (see Figure 1; here and in the remaining illustrations, we present 24-hour data recorded on January 15, 2022, the day of the Hunga Tonga volcanic eruption). Owing to the global reach (low attenuation) of ELF waves, a single measuring station can monitor thunderstorm activity worldwide within this frequency range, distinguishing different thunderstorm regions based on azimuths of recorded waves. Such measurements were typically conducted on a limited scale by analyzing individual impulses from the strongest thunderstorm discharges. In this study, we propose a new method that utilizes ELF magnetic signals across the full available range of wave amplitudes. We demonstrate how disturbances at 50 Hz from the power grid can be filtered out from measurement data, enabling the azimuth determination of waves using relatively low-amplitude impulses. This method has been applied to measurements at 3 kHz frequency from our Hylaty station in Poland, allowing for continuous monitoring of thunderstorm activity in major active thunderstorm regions on Earth. It is important to note that our measurements of only two perpendicular magnetic components of the propagating wave allow for determining the propagation direction but not its orientation. Thus, the azimuths of impulses have an ambiguity of ±180 degrees, as illustrated in Figure 2, with showing only partial symmetry between azimuths separated by 180 degrees.
The approach proposed in this study is based on determining the azimuth of incoming waves from differential magnetic field measurements ∆BNS and ∆BEW, derived from successive measurements of the wave's magnetic field, here at scales shorter than the duration of individual impulses. Previously, considerably more complex modeling of such impulses was required to determine azimuths, which entailed not only substantial effort but also limited the utilization of measurement time without strong impulses. The proposed differential method, with selected analysis parameters (n, rmin, rmax), allows the azimuth of the wave reaching the sensor at a given moment to be determined, where the parameter n defines the time interval between considered measurements, and rmin and rmax define the minimum and maximum amplitude for the analyzed field variations, √(∆BEW2 + ∆BNS2). At n=1, the azimuths in the ELA11 detector correspond to time intervals of 0.33 ms. A measured impulse from a single discharge can last several milliseconds, and with the global discharge frequency estimated at 50-100 per second, overlap of different impulses in the measurements is limited, suggesting the potential of the method for reliable azimuth measurements from different storms. The method proves highly effective, enabling the separation and tracking of signal azimuths from multiple storms in seconds' time scales (see Figures 2, 3, and 4), as well as partial separation of nearby and distant impulses, owing to the high dynamic range of the applied magnetic sensor and the wide amplitude range of ELF impulses analyzed within a single procedure. This opens new research possibilities for both thunderstorm phenomena and ionospheric variability.
Volcanic eruptions also produce electrical discharges similar to thunderstorm discharges (see Figure 1). The eruption of the Hunga Tonga volcano in January 2022 was notably active in this regard. The strong point-like source of ELF signal from this eruption allowed us to measure the azimuth deviation of the signal as it propagated from the Pacific to Poland through the polar region, partially propagating near the solar terminator, and to demonstrate how our method enables separate analysis of highly refracted ionospheric signals from Hunga Tonga, arriving at our measurement station from over 16 Mm away, from nearby thunderstorm signals with the same azimuth (compare Figures 2, 3, and 4).
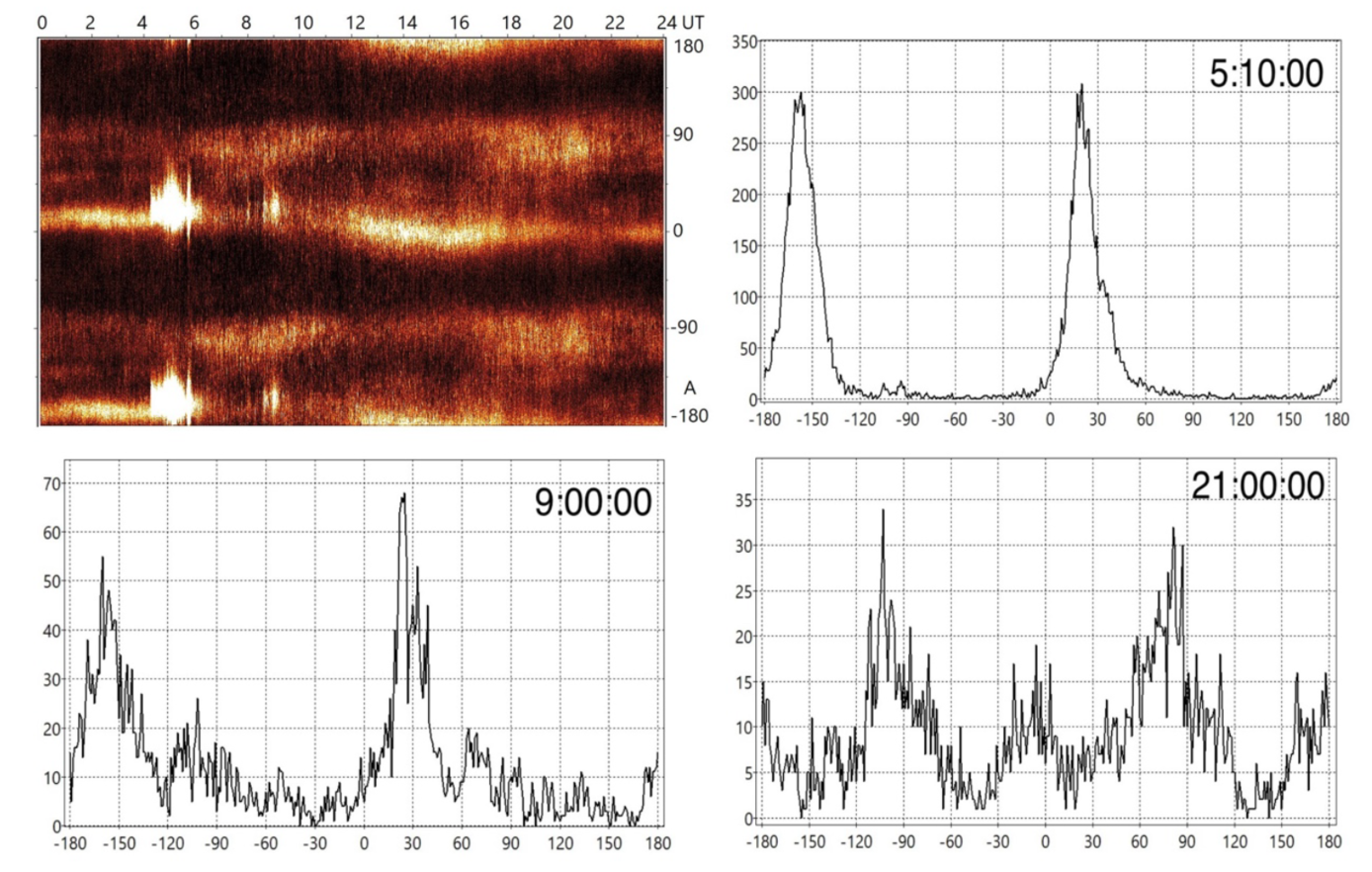
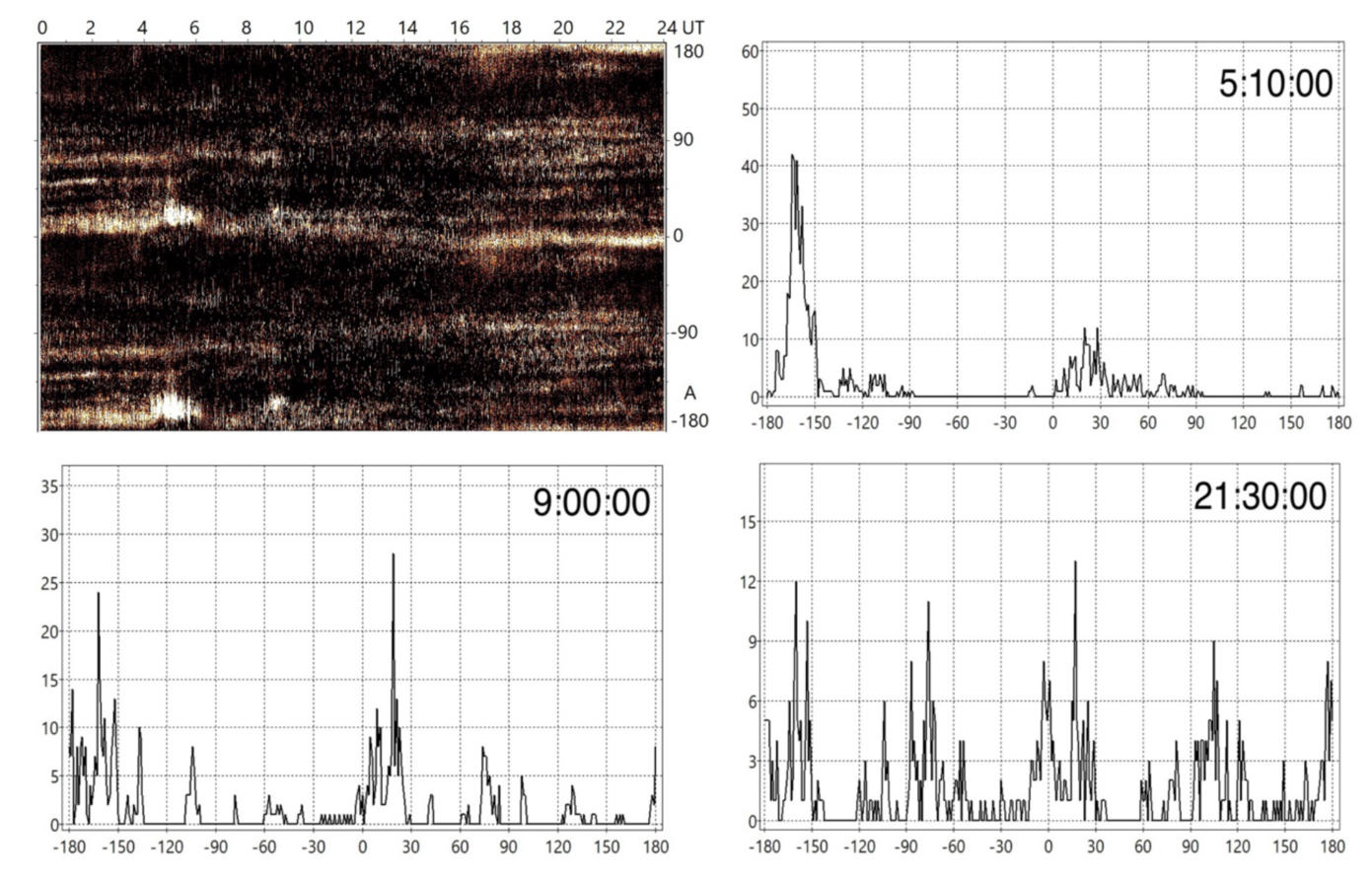
Original publication: Kubisz, J., Gołkowski, M., Mlynarczyk, J., Ostrowski, M., Michalec, A., New Method for Determining Azimuths of ELF Signals Associated With the Global Thunderstorm Activity and the Hunga Tonga Volcano Eruption, Journal of Geophysical Research: Atmospheres, 129, article id. e2023JD040318 (2024).
The study discussed here was conducted by an international research team from Jagiellonian University, AGH University, and the University of Denver, Colorado, led by Jerzy Kubisz from the Astronomical Observatory.
Jerzy Kubisz Astronomical Observatory Jagiellonian University Jerzy.Kubisz [@] uj.edu.pl |
Michał Ostrowski Astronomical Observatory Jagiellonian University Michal.Ostrowski [@] uj.edu.pl |